Tolerancing 0ptimization Strategy #2 (Combination of Linear and Geometric)
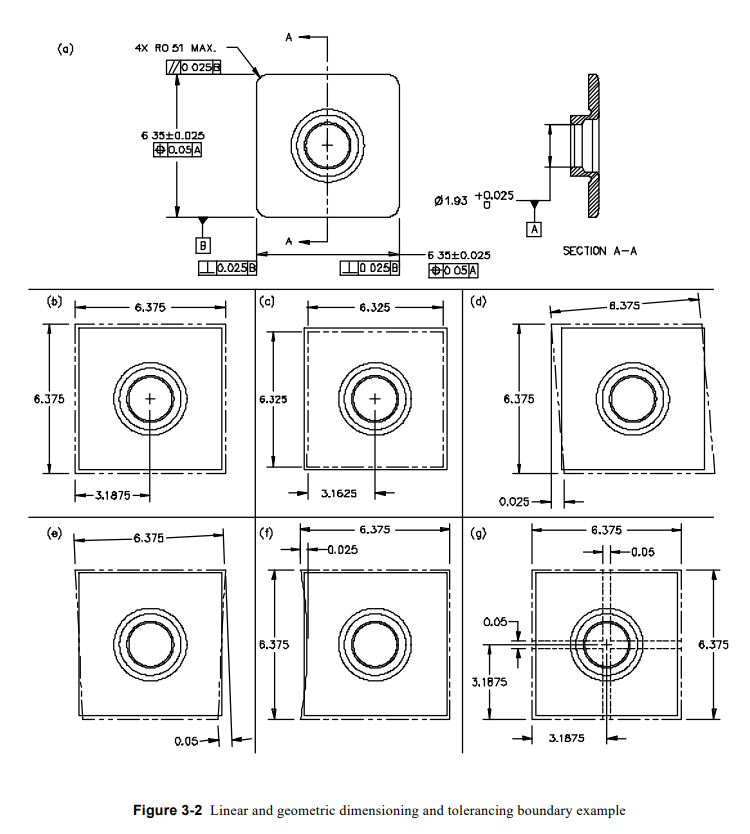
Fig. 3-2a is a combination of linear and geometric callouts, and clearly adds controls for orientation of one surface to another. This is achieved with perpendicularity callouts on the left and right sides of the part in relationship to datum -B-, along with a parallelism callout on the top of the part, also to datum -B-. In addition, position callouts were added to each of the size dimensions (6.35 mm ±0.025 mm) and were controlled in relationship to datum -A-, which is the “axis” of the inside diameter (1.93 mm +0.025 mm /–0 mm).
Figs. 3-2b to 3-2g define some of the conditions allowed by these drawing callouts.
Fig. 3-2b shows a part perfectly square and made to its maximum size based on the specification (6.375 mm), which would be an acceptable part for size. Assuming the hub was exactly in the center where
the designer would like it to be, this part would measure 3.1875 mm. Unlike the negative impact mentioned in regards to Fig. 3-1b, this measurement adds no negative impact to specifications because the “center plane” is now being located from the “center” of the inside diameter.
Like Fig. 3-2b, Fig. 3-2c shows a part that is perfectly square and made to its minimum allowable size based on the specifications (6.325 mm), which is again acceptable for size. Again, assuming the hub was
exactly in the center where the designer would like it to be, the 3.1625 mm measurement has no negative impact on specifications.
Fig. 3-2d (like Fig. 3-1d) shows a part on the large side of the tolerance allowed, with its orientation skewed to the shape of a parallelogram. In this example, however, the perpendicularity callouts added in
Fig. 3-2a control the amount this condition can vary. In this case it is 0.025 mm. The problem that stands out here is that the designer’s original intent stated: to have the external boundary utilize a space of 6.35mm ±0.025 mm “square.” Based on this requirement, it’s clear this objective was not met. Granted, it is controlled tighter than the requirements defined in Fig. 3-1a, but it still does not meet the designer’s expectations.
Fig. 3-2e shows a combination of Figs. 3-2b and 3-2c (like Figs. 3-1b and 3-1c), in that it allows the shape to be small at one end and large at the other. Unlike Figs. 3-1b and 3-1c, Fig. 3-2e restricts the
magnitude of change from one end to the other by the parallelism and perpendicularity callouts shown in Fig. 3-2a.
Because this part is symmetrical, a unique problem surfaces in this example. Using Fig. 3-2e, assuming the bottom surface is datum -B-, the top surface is shown to be perfectly parallel. Due to the part being
symmetrical, it is impossible to determine which surface is truly datum -B-. So, if we assume the left-hand edge of the part as shown in Fig. 3-2e was the datum, the opposite surface (based on the shape shown)
would show to be out of parallel by 0.05 mm.
This clearly shows that problems in the geometric callouts are not only in the design area, but also in the ability to measure consistently. Like-type parts could measure good or bad, depending on the surface identified as datum B
Fig. 3-2f again shows displacement in shape allowed. In this case it shows a part that is for the most part large, except all the variability (0.025 mm) shows up on one edge. The limiting factor (depending on
which surface is “chosen” as datum -B-) is the perpendicularity or parallelism callouts.
Fig. 3-2g is showing a part made to its large size (like Fig. 3-1b), and the 0.05 mm zone allowed by the position callout. Unlike Fig. 3-1g, the larger or smaller size of the square shape has no impact on the
position. Based on the callout in Fig. 3-2a, the center planes (mid-planes) in both directions must fall inside the dashed boundaries.
The above comments concerning Fig. 3-2a are intended to show a tolerancing strategy that encompasses both liner and geometric callouts but still does not meet the designer’s intended expectations. Based on this, the designer modified the drawing again, as shown by Fig. 3-3a, which led to strategy #3.
Tolerancing 0ptimization Strategy #3 (Fully Geometric)
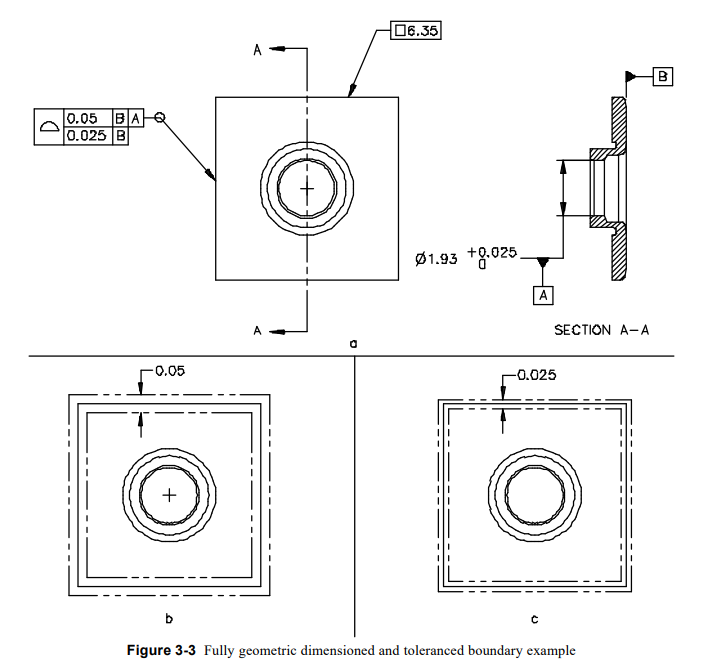
Tolerancing 0ptimization Strategy #3 (Fully Geometric)
Fig. 3-3a is the optimum dimensioning and tolerancing strategy for this design example. In this case, the outside shape is defined clearly as a square shape that is 6.35 mm “basic,” and is controlled with two
profile callouts. The 0.05 mm tolerance is shown in relationship to datums -B- and -A-, controlling primarily the “location” of the hub in relation to the outside shape (depicted by Fig. 3-3b). The 0.025 mm tolerance is shown in relationship to datum -B- and controls the total variation of “shape” (depicted by Fig. 3-3c).
This tolerancing strategy clearly defines the designer’s intent.
Link for Tolerance Optimization Strategy 1
https://udhasulnayakblog.com/tolerancing-optimization-strategies-design/
Link for General Motor Engine Assembly line LM-850
https://youtu.be/fjCf9jXXp0o
Check link for Tolerance Stack Up Analysis
https://youtu.be/0LVnLDltRC0